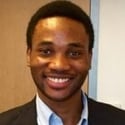
Viruses, however pathogenic, have propelled scientists to relentlessly investigate their modus operandi, so as to use them for beneficial purposes such as gene therapy. Gene therapy is being pursued as a treatment option for a variety of diseases and disorders, such as hemophilia and certain cancers. It is theoretically very lucrative, due to the fact that such therapy could lead to a long-lasting solution or cure for the disease/disorder being treated. The basic underlying principle of gene therapy is gene editing—i.e. making changes to the diseased cells and their progeny.
Gene editing entails correcting a gene at a specific locus. Gene transfer, on the other hand, is the incorporation of a full correct copy of a mutated gene into the genome. Tools used in gene editing include restriction enzymes, CRISPR/Cas, Zinc finger nucleases, and Meganucleases, just to name a few. These enzymes either contain domains that recognize specific sequences of DNA, or can have guide sequences that find the complementary DNA strand to be severed. Based on outcome and specificity required, scientists use these tools to introduce double-strand breaks (DSB) at regions of interest and provide corrected DNA strands with homologous arms that flank both sides where the endonuclease activity occurred, thus allowing the cell’s homology repair system to integrate the correct DNA sequence into the genome.
Each gene editing tool comes with its limitations—e.g. the CRISPR/Cas9 RNA guide requires a protospacer adjacent motif (PAM) before binding can occur with its target DNA. The PAM sequence is 5’-NGG-3’, where the N represents any nucleotide. The Cas9 endonuclease, upon binding its target DNA, severs approximately 6-8 nucleotides upstream of the PAM sequence. Hence, the prerequisites for a beneficial CRISPR/Cas9 system highlights obstacles that hinder their applicability with regard to precision. Nevertheless, more is still being learned about this relatively new field of engineered endonucleases. The CRISPR/Cas9 system has already been successfully re-engineered to perform nicks (cutting just one strand), opposed to a DSB.
Gene transfer is mainly conducted with viral or bacterial (plasmid) vectors. Of note is the use of retroviruses, which seek to ensure a permanent integration of the new corrected gene within a significant amount of cells in the tissue or organ being targeted for treatment. A classic example of genome editing with gene transfer is the gene therapy clinical trial in patients with Severe Compromised Immunodeficiency (SCID), where scientists used a gamma retrovirus and transduced the patients’ hematopoietic stem cells (HSCs) with success in “full correction of the disease phenotype,” which is the presence of a defective cytokine receptor that prevented proper differentiation of NK cells and T-cells—hence, patients have an almost, if not completely, non-functional immune system.
Cells were grown in factors that stimulated cell division (since gamma retroviruses infect dividing cells only) and then transduced with a viral vector possessing the wild type cytokine receptor gene. Unlike plasmids, wherein promoters are necessary for gene expression, the viral vector is armed with its inherent promoter, the long terminal repeat (LTR) regions, which flank the viral 5’ and 3’ terminals. Due to the fact that retroviral integrations into the genome are random, their intended use could lead to catastrophic consequences, such as leukemia. This is because the virus might integrate near oncogenes, leading to their overexpression via the LTR.
Gene editing, along with bone marrow transplantation, has gone a long way to instill hope in mankind’s battle with disease. Take, for instance, one of the greatest medical accomplishments yet, “The Berlin Patient,” who was cured of HIV and leukemia (which he developed later) via bone marrow transplantation. The discovery of a T-cell receptor (CCR5), which is required for the most prevalent strain of HIV (R5) to enter into the cell and replicate, has led researchers to investigate how to disrupt the CCR5 gene with editing tools such as CRISPR/Cas9. Moreover, some people are null for the CCR5 gene—hence, cannot get infected with HIV—making transplantation of their bone marrow into patients a lucrative prospect. However, obstacles such as grafts-versus-host disease still stand in the way, and personalized treatments are often needed.
Although bioengineering technology is rapidly advancing, much more work needs to be done—especially regarding the study of retroviral integration sites (RIS) and how viral manipulation can lead to locus-specific gene transfer, as well as how to harness the ever-evolving applicability of gene editing techniques. The adverse effects that could come about with viral gene therapy have left scientists and researchers actively seeking for alternative ways to bring about life-saving treatment. This search has brought about the CRISPR/Cas9 endonuclease, which was recently used in a clinical trial in China to treat a patient with aggressive lung cancer. Nonetheless, there are still plenty of hurdles to overcome as we strive to master the art and science of gene therapy.
Quartzy is the world’s No. 1 lab management platform. We help scientists easily organize orders, manage inventory, and save money. We’re free and always will be. Visit Quartzy.com or reach out at info@quartzy.com.
Interested in writing for The Q? Send us an email!
Share this:
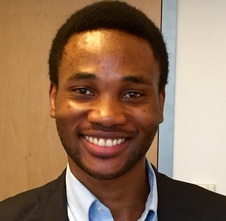
Flavio Somanji
Flavio holds a BS degree in Biology and Neuroscience from Syracuse University and is a member of the research staff at Tufts University School of Medicine. He is concurrently pursuing a Masters degree in Bioengineering and Nanotechnology at Harvard University. Flavio spends his spare time by applying inherent entrepreneurial skills in the tech startup world which has led to the establishment of EcoDemand™, a soon-to-be launched ecommerce marketplace.