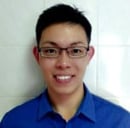
Biomedical engineering is a highly interdisciplinary field that integrates knowledge from physics and chemistry to solve biological problems. Although it is becoming an extremely popular field and biomedical jobs are expected to increase substantially, due to its diverse research topics, many people do not fully understand what it comprises. This article is part of a series that explains the various subject matters within biomedical engineering. Through this series, I hope to improve understanding of this field, and potentially help students who are choosing majors and contemplating graduate education make their decisions. This first spotlight is on neural engineering.
What is neural engineering?
Traditional neuroscience focuses on neurobiology and neurochemistry, where researchers investigate the effects of genes and chemical molecules, such as hormones, on neuronal functions. However, in recent years, researchers have begun to appreciate the effects of physical forces, such as mechanic (i.e. shear stresses and tensions) and magnetic stimulation on neuronal activities. Neural engineering is a sub-branch in biomedical engineering that aims to unravel the way physical forces interact with neurons.
What are some tools for neural engineering?
Microfluidics chamber
In 2005, Taylor and colleagues made used microfluidic chambers to isolate different components of neurons—i.e. cell bodies from axon and neurite extensions (axons and dendrites). With this device, scientists can apply specific stimulation to different parts of neurons to observe differences in response. This is crucial, as the functions of neuronal substructures are known to be highly diverse. For instance, certain ion channels are preferentially expressed at the axonal boutons where synapses are formed with dendritic spines. Furthermore, when coupled with physical forces such as shear stresses, researchers can also make use of microfluidic devices to observe how neurons react to blood flow, which is useful in devising strategies to enhance permeability through the blood-brain barrier for drug delivery applications.
Magnetic nanoparticles
Although microfluidic chambers allow researchers to better target different neuronal structures, the spatial resolution is still not as high as using magnetic nanoparticles. Using antibody-coated magnetic nanoparticles, researchers can target membrane proteins that have differential expressions on neuronal cell bodies, axons, and dendrites. With this class of technique, researchers have managed to extend axons using mechanical forces provided by magnetic nanoparticles under magnetic manipulation. This finding has implication for promoting axonal regeneration following injury. Recently, Tay and coworkers also made use of magnetic nanoparticles for remote induction of calcium into neural networks.
What can neural engineering achieve?
There are a few areas that neural engineers are most actively pursuing.
Directing the growth and migration of neurons
Researchers have found that by synthesizing materials with parallel fibers, they can bias the direction in which neurons grow their neurites. This research has application in spinal cord injury repair, where there is a lack of functional neuronal connection between regions up- and downstream of the injured site.
Magneto-genetics to modulate behaviors
In this technique, neurons are genetically modified to biomineralize magnetic nanoparticles that bind to membrane ion channels that are sensitive to mechanical stimuli. Upon magnetic stimulation, the ion channels open, allowing calcium influx that modulates downstream neuronal functions. Using this technique, scientists have demonstrated the ability to remotely control feeding behaviors.
Neural interfaces
This area of research encompasses enhancing or restoring neuronal functions with engineered devices. Some examples would include computer-brain interfaces, microsystems to deliver chemical, optical, electrical or magnetic stimuli to neurons, and neural prostheses. One of the key challenges in this field is to match the mechanical (and immunological) compatibility of brain tissue (which is extremely soft) and invasive interface implants, which tend to be stiff. Due to investments in brain research, such as the U.S. BRAIN initiative, there have been recent innovations, such as using flexible mesh electronics for invasive large-scale, single-neuron recording. The National Institutes of Health is also funding technologies to improve the spatial and temporal resolution of non-invasive magnetic stimulation and recording from the brain to improve our understanding of brain circuitry and diseases.
Opportunities
- The S. National Institute for Mathematical and Biological Synthesis organizes meetings/conferences to bring together scientists who are interested in using mathematical models on biological system. One of the past sessions focused on applying mathematics to understand neurite growth.
- Janelia Farm organizes the annual Genetic Manipulation of Neuronal Activity meeting, and provides generous travel fellowships on top of free registration, lodging, and food for all accepted participants. The meeting is also intentionally small to provide junior-level scientists with ample opportunities to interact with senior scientists.
- NSERC-CREATE in Canada holds an annual workshop for scientists in neural engineering. This center has a special focus on axon guidance mechanisms and formation of new synapses.
Quartzy is the world’s No. 1 lab management platform. We help scientists easily organize orders, manage inventory, and save money. We’re free and always will be. Visit Quartzy.com or reach out at info@quartzy.com.
Interested in writing for The Q? Send us an email!
Share this:
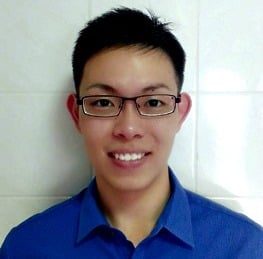
Andy Tay
Andy Tay is a graduate student in the University of California, Los Angeles and an instructor in the National University of Singapore. His research focuses on magnetic neural stimulation and magnetotactic bacteria. He enjoys science communication and using the gym in his free time.