The Polymerase Chain Reaction, PCR, is one of the cornerstones of molecular biology. It allows the selective amplification of a target DNA sequence that occurs only once within a large and complex DNA structure such as genomic DNA. PCR is composed of three temperature-dependent steps: (1) denaturation of the double-stranded template DNA into two single strands; (2) annealing of two synthetic oligonucleotide primers to the target DNA sequence; and (3) extension, where a thermostable DNA polymerase catalyses the synthesis of two daughter DNA molecules starting at the 3’ end of the annealed primers.
Many factors can affect the efficiency and specificity, and hence the success of PCR. Of these, the design of the olignucleotide primers is of the utmost importance. Primers should be designed in a manner that ensures high yield and specificity (no mispriming or amplification of unwanted sequences), and takes into account the downstream application of the amplification product. For example, if the PCR product is intended for cloning, the primers should include the appropriate restriction sites (link to molecular cloning part 1 post) at their 5’ ends. Whenever designing primers, always make sure that the open reading frame of the gene in interest is maintained! Below are some considerations for successful primer design:
- Length: Oligonucleotide primers are usually 18-30 nucleotides in length, long enough to specifically bind to their target, and short enough to decrease the probability of secondary structure formation. Primers should have a 15 bp target overlap at the 3’ end.
- Base composition: Aim for an equal number of A, T, C, G, and a %CG of 40-60% to ensure stable binding of primer and template. Avoid dinucleotide repeats (e.g. ATATATAT) and long runs (>4) of a single base. Both of these can lead to mispriming.
- CG clamp: Include a GC clamp at the 3’ end within the last five bases. The hydrogen bonding between G and C bases is stronger than between A and T bases, and the presence of a GC clamp will promote tight binding at the 3’ end of the target sequence. However, do not include more than 3 G’s or C’s within the last five bases at the 3’ end because of the higher probability of primer-dimer formation (amplification of the primers themselves).
- Restriction sites: Restriction enzymes cut poorly at terminal sites. Therefore, if adding a restriction site at the 5’ end of your primer, make sure to include an additional 6-8 bp (e.g. GCGGCG-restriction site-your sequence) to increase cleavage efficiency.
- Start codon/Kozak sequence: Include a start codon (ATG) in the forward primer. Additionally, if the PCR product will be cloned into a mammalian expression vector (e.g. pcDNA 3.3), make sure to include a Kozak sequence in the forward primer, unless the sequence is included in the cloning vector. Include a stop codon (TAA is preferred) in the reverse primer. You can use 2 stop codons in series to increase the efficiency of termination.
- Melting temperature: Melting temperature (Tm) is the temperature at which 50% of the primer has annealed to the template, and is an indication of the stability of the DNA duplex. The specificity of PCR depends strongly on the melting temperature (Tm) of the primers. Try to design primers with similar Tm’s (within 5C of each other) and between 60 and 75°C.
- Annealing temperature: Ideally, although not always possible, you should use an annealing temperature (Ta) that is about 5°C below the lower of the two primer Tm’s. If the Ta is too low, the likelihood of base pair mismatches and/or partial annealing will increase. This will cause one or both primers to anneal to non-target sequences and result in non-specific products. Conversely, a Ta that is too high will significantly reduce the likelihood of primer-template annealing, leading to a decrease the PCR product yield. When my PCR doesn’t work, the first parameter I change is the annealing temperature.
- Hairpin formation: A hairpin is formed by intramolecular interaction within the primer, and should be avoided. Stable hairpins, indicated by a large negative value for ∆G (the energy required to break the secondary structure), should be avoided, particularly at the 3’ end. ∆G values of -3 and -2 Kcal/mol are tolerated internally and at the 3’ end, respectively. Avoid hairpins with a melting temperature close or higher than that of the primer.
- Self-dimers: If a primer is homologous to itself, intermolecular interactions between same sense primers will form, possibly more readily than primer-template hybridization, thus reducing the product yield. ∆G values of -6 and -5 Kcal/mol are tolerated internally and at the 3’ end, respectively.
- Heterodimers: These are formed by intermolecular interactions between the forward and reverse primers at regions where they are homologous, and will also decrease the product yield by competing for DNA polymerase and dNTPs. ∆G values of -6 and -5 Kcal/mol are tolerated internally and at the 3’ end, respectively.
- Cross homology: Primers designed to amplify a specific sequence must not amplify other sequences in the mixture. After designing your primers, submit them to a BLAST search to test their specificity.
Web-based tools
Many primer design tools are available and can greatly help in reducing the cost and time spent in experimentation and troubleshooting. Here are my favorites:
- IDT OligoAnalyzer 3.1 Input your primer or primers pairs, and the tool will analyze them for base composition, GC content, melting temperature, hairpin formation, and self- and cross- dimerization.
- Primer-BLAST allows users to design new target-specific primers in one step as well as to check the specificity of pre-existing primers.
Quartzy is the world’s No. 1 lab management platform. We help scientists easily organize orders, manage inventory, and save money. We’re free and always will be. Visit Quartzy.com or reach out at info@quartzy.com.
Interested in writing for The Q? Send us an email!
Share this:
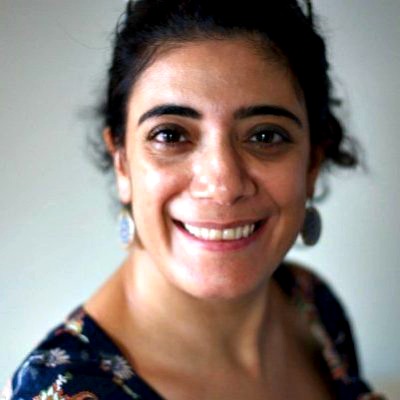
Daad Abi-Ghanem
Daad studied avian immunology at Texas A&M University. She is director of R&D at a biotech company in Portland, Oregon. She enjoys communicating hands-on lab experience, reading, writing, running, hiking, and crossword puzzles.