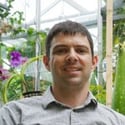
CRISPR! It just works. Like magic. At least that's how it’s often portrayed. It is a powerful gene-editing technology, no question. Like any experiment, however, it requires specialized materials, effort, and workflow design, too. Lastly, there’s the matter of actually ending up with stable CRISPR-Cas9 induced changes to a targeted sequence of DNA. New applications for CRISPR-Cas9 technology are being developed, and new Cas9-like enzymes are being discovered, creating more possibilities, but also more decisions. The recent CRISPR-Cas9 patent ruling is unlikely to be the last iteration of the technology to be fought over.
Different study systems/organisms also open up or constrain possibilities of CRISPR-Cas9 application. As I’m most familiar with plants (and CRISPR methods for them), I will use them as a case study.
In plants, transformation efficiency (the delivery of the CRISPR-Cas9 system into the plant) is low relative to stably transforming other sequences of DNA into plants, due to its large size. The most common transformation vector to create transgenic plants, Agrobacterium tumefaciens, is also variably efficient, depending on plant species targeted. Multi-site targeting was initially a bit challenging in plants, as each target increased transgene size. However, one clever method is introducing polycistronic guide RNAs (gRNAs) with endogenous, nuclease cleavable, tRNA sequences as small spacers, allowing more CRISPR-Cas9 targets per transgene. A plus in plants is that off-target effects in plants are rare. So once it’s in the plant, CRISPR-Cas9 works well—at least on plants studies thus far.
Verifying any heritable and stable mutations is a key goal of most CRISPR-Cas9 editing. This isn’t so difficult for a single target, but introducing multiple targets complicates screening if all are needed in a single plant, or if all the different permutations of the targets are needed for an experiment. Usually, finalized edited plants also require segregating out the CRISPR-Cas9 transgene. For screening a small number of potentially edited genes, sequencing pools of second-generation plants containing the CRISPR-Cas9 transgene and seeing if mutations in target regions are present via direct sequencing can pull out candidate lines containing mutations.
Another identification method is whole-genome sequencing. This is a good way to identify all the changes in a genome, and can readily identify any off-target effects. The other way to guard against off-target effects is getting multiple independent lines carrying edits in the same gene (in genetics, multiple alleles showing similar results is always good). Whole-genome sequencing is most useful for CRISPR/Cas9 screens available in some systems (not plants, though) that involve many random targets. They involve finding a phenotype deviating from the wild type, and then finding the edited target gene of that cell line through whole-genome sequencing.
Screening through mutations is a lot of work. One CRISPR innovation generated in the plant-science world is to flank guide RNAs (gRNA) with self-cleaving ribozymes. Once the RNA is expressed, the ribozymes cleave themselves away, leaving the free gRNA to pair with the Cas9 enzyme. This increases the CRISPR-Cas9 system’s flexibility, so the gRNA can be driven by any promoter, instead of the standard U3 or U6 Pol III-dependent promoters, and enables use of CRISPR-Cas9 as an in-vitro diagnostic assay. In-vitro addition of Cas9 and a self-processing ribozyme flanked gRNA to a PCR product of a targeted region will result in cutting if the sequence hasn’t been edited, or not cutting if it has, thus making screening targets easier.
Another tool for screening small insertions/deletions is a tool originally developed to easily assay point mutations or frameshifts. CAPS (Cleaved Amplified Polymorphic Sequences) and its clever cousin, derived CAPS (dCAPS), can be used to track an already known mutation. First, sequencing to define the target is needed, and then a marker using a restriction enzyme-based difference can be developed to track the mutation induced by the CRISPR-Cas9 system.
CRISPR can, of course, do more than insertion/deletion events, though that is beyond the scope of this article. However, similar principles of experimental design and effort still apply.
CRISPR is evolving. More than one bacteria has a CRISPR-Cas9-like system in its genome. One that’s newly being applied is CRISPR-Cpf1. From a different bacteria, Cpf1 works similarly to Cas9 but has different target restrictions, requires smaller gRNAs, and, at least when used in rice, is highly efficient at editing intended targets. Cpf1 cuts DNA slightly differently than Cas9, too. Likely, other enzymes exist in nature to be discovered and applied in myriad ways to edit genomes. CRISPR is this century’s restriction endonuclease, and a great addition to the molecular biology toolbox.
But remember: Even with a seemingly magical tool like CRISPR-Cas9, it is still science—and that means design, effort, and thought be put into experiments.
Quartzy is the world’s No. 1 lab management platform. We help scientists easily organize orders, manage inventory, and save money. We’re free and always will be. Visit Quartzy.com or reach out at info@quartzy.com.
Interested in writing for The Q? Send us an email!
Share this:
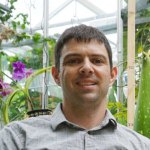
Ian Street
.